Have you ever wondered how our solar system came into existence? How did our whole solar system get started? Through something that we call a solar nebula. But what is a solar nebula? A massive cloud of hydrogen gas and dust that set the stage for everything we see today. About 4.6 billion years ago, a huge, messy, immense cloud collapsed under its gravity. That’s the solar nebula. It flattened into a spinning disk, and most of the material gathered at the center formed the Sun. The remaining dust and gas eventually turned into our solar system’s planets, moons, and other objects.
Check this article out, too: What is a Wormhole? Your Ultimate Guide to Wormholes
This formation process wasn’t just a random event—it was a good mix of gravity and astrophysics. That’s why knowing what is a solar nebula, its history, origins, and how it worked is important in astronomy and astrophysics. After all, it shaped our current universe.
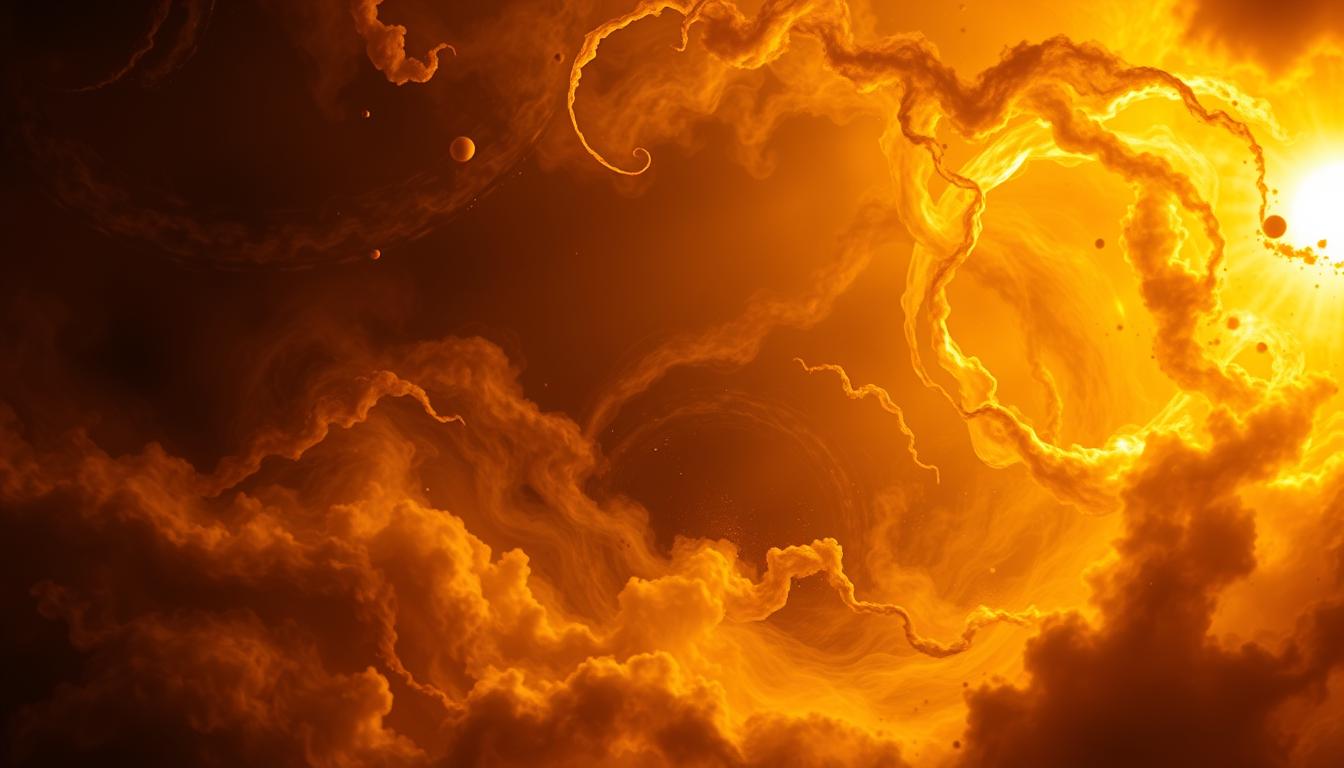
Introduction to the Solar Nebula and Early Star Formation
Let’s rewind the cosmic clock to way back—like over 4.5 billion years ago. When even the stars haven’t been formed yet, let alone our Sun, Earth, or the Wi-Fi. Just a huge cloud of dust and gas hanging out in space, waiting for something exciting to happen. That cloud – the Solar Nebula – was the beginning of everything. It had one job: turn into a star (and a few planets on the side). This is where things get interesting. With a little push (more on that below), this calm, chilly cloud started to move. Over time, gravity pulls these particles together, creating dense regions called molecular clouds. Inside these clouds, the magic of star formation begins. That’s how stars and solar systems like ours get their start.
Origins in Interstellar Dust and Gas
The solar nebula started out as part of the interstellar medium. A fancy way of saying it was just a chunk of the space fog of dust and gas floating around our galaxy. This stuff was everywhere, leftover from older stars that exploded and sent their bits flying across space. Interstellar dust and gas are the raw materials for stars. These tiny particles, often just a fraction of a millimeter in size, clump together under the influence of gravity. This process, called accretion, is the first step in creating a star.
We’re talking about hydrogen, helium, and tiny specks of solid stuff like carbon and silicon. It wasn’t dense or warm or special. These materials are like the flour and sugar in a star recipe. When they are alone, they’re not worth much. With the right conditions, they create stars.
The Role of Gravitational Collapse
Here’s where gravity comes in and says, “I got this.” Gravity is why our big fluffy space cloud started falling in on itself. Maybe a nearby star exploded and gave it a little nudge, or maybe it just got too heavy to stay spread out. Either way, gravity took over.
Gravitational collapse is the key process in star formation. When a cloud becomes dense enough, its gravity causes the star to collapse towards itself. This collapse speeds up over time. It creates a hot, dense core that will eventually become a star. The core pulls in more gas and dust from the surrounding disk as it grows. This continues until the star reaches its full size. The gravity and rotation shape the final structure of the star and its surrounding system.
What is a Solar Nebula?
Let’s break it down nicely and easily: a solar nebula is the big, messy cloud of space from which our solar system comes. It’s the blueprint. The cosmic starter pack. Scientists use the term “solar nebula” to describe the giant cloud of gas and dust that collapsed under its own gravity and eventually became the Sun and all the planets around it. In modern astronomy, the nebula is a circumstellar disk of gas and dust. It’s the birthplace of stars and planets, including our Sun. This model has come a long way since the days of Kant and Laplace, who first proposed the idea of a spinning disk. Historically, the nebula was seen as a simple cloud of material. Today, we understand it as a dynamic, spinning disk.
The components of the nebula—primarily gas and dust—play a crucial role in star and planet formation. These materials clump together under gravity, forming the building blocks of anything in space.
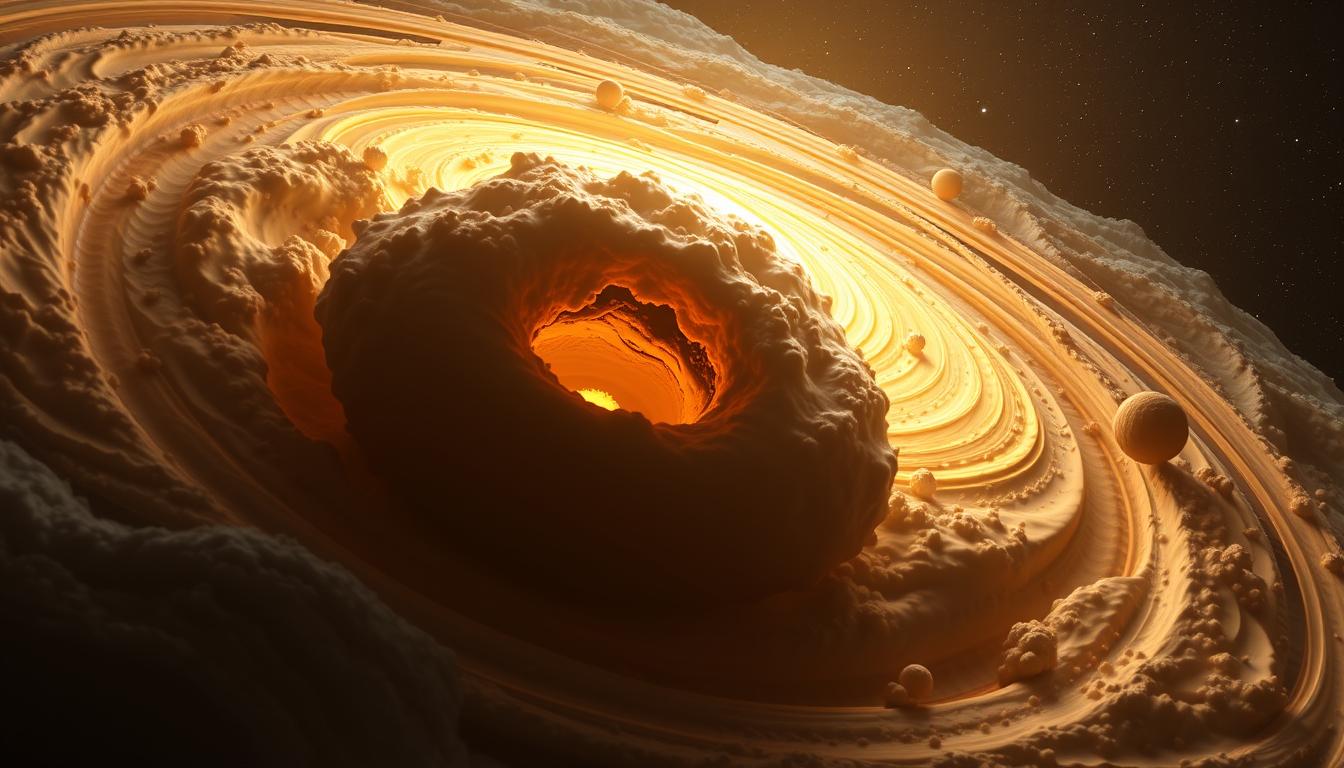
The Nebular Hypothesis and Its Historical Evolution
Alright, now let’s give a shoutout to the space nerds of the past who actually figured all this out without telescopes that tweet pictures from Mars. The idea that stars and planets come from collapsing clouds has been around for centuries. We call it the nebular hypothesis.
Back in the day, Kant and Laplace first proposed this theory. It was basically an observation at the time, but they laid the groundwork. Kant and Laplace envisioned a spinning cloud of gas and dust that collapsed under gravity. Their model explained how the Sun and planets formed from this primordial material. Their ideas were groundbreaking, and they were just beginning a long journey of discovery.
Contributions of Kant and Laplace
Kant’s work in the 18th century introduced a new idea. It was the rotating cloud that flattened into a disk. Laplace later refined this model. He suggested that the Sun formed at the center while planets formed from the material around them. Their combined efforts provided the first coherent explanation for the structure of our solar system. These early theories were based on philosophical reasoning rather than direct evidence. They didn’t have space probes or fancy simulations—just brains, telescopes, and imagination. Even though parts of their theory weren’t 100% right, their basic idea stuck: stars and planets form from spinning clouds of gas and dust.
Modern Adaptations in Planetary Science
Fast forward a few centuries, and we’ve got high-powered telescopes, satellites, and computers doing all the heavy lifting. Scientists today have taken the original nebular hypothesis and refined it into a full-on science of planetary formation. These advancements have transformed the nebular hypothesis from a descriptive theory to one supported by observational evidence.
Now we know about things like accretion disks, protoplanets, and how different elements behave in young solar systems. Modern planetary science builds on the foundation that Kant and Laplace laid down. The good thing now is that we don’t have to guess as Kant and Laplace did. We can use more actual data and eliminate guesswork.
Accretion Processes: From Dust Grains to Planetesimals
Okay, now we’re getting into the good stuff. What sparked the transformation of tiny dust particles into massive planets? The answer lies in accretion, a multi-stage process that shapes everything. This is just a fancy science word for “stuff sticking together.” Like a snowball rolling down a hill and picking up more snow as it goes.
It all began with microscopic grains of dust floating in the early system. Initially, these particles clumped together because of static electrical forces. Ever rubbed a balloon on your hair and watched it stick to the wall? Same idea. These little grains used their electric charges to attract each other. As these clumps increased in mass, their gravitational pull became stronger. This is when static forces leave their work to gravity. This early collection was the first step in building larger bodies. Over time, these tiny clumps grew into kilometer-scale objects that we call planetesimals.
From Planetesimals to Proto-Planets
Once these clumps got big enough and became planetesimals, they had enough gravity to pull in more stuff and grow even bigger. The stronger and bigger the gravitational pull, the more stuff is coming your way. This stage of accretion is extremely critical in forming the next level. We call it proto-planets. These are early versions of planets that were on their way to becoming the worlds we know today, like the evolution here on Earth. One exciting thing about the change to proto-planets is that the observational data supports this model. For example, we can see the chemical signatures of early formation processes in meteorite analysis.
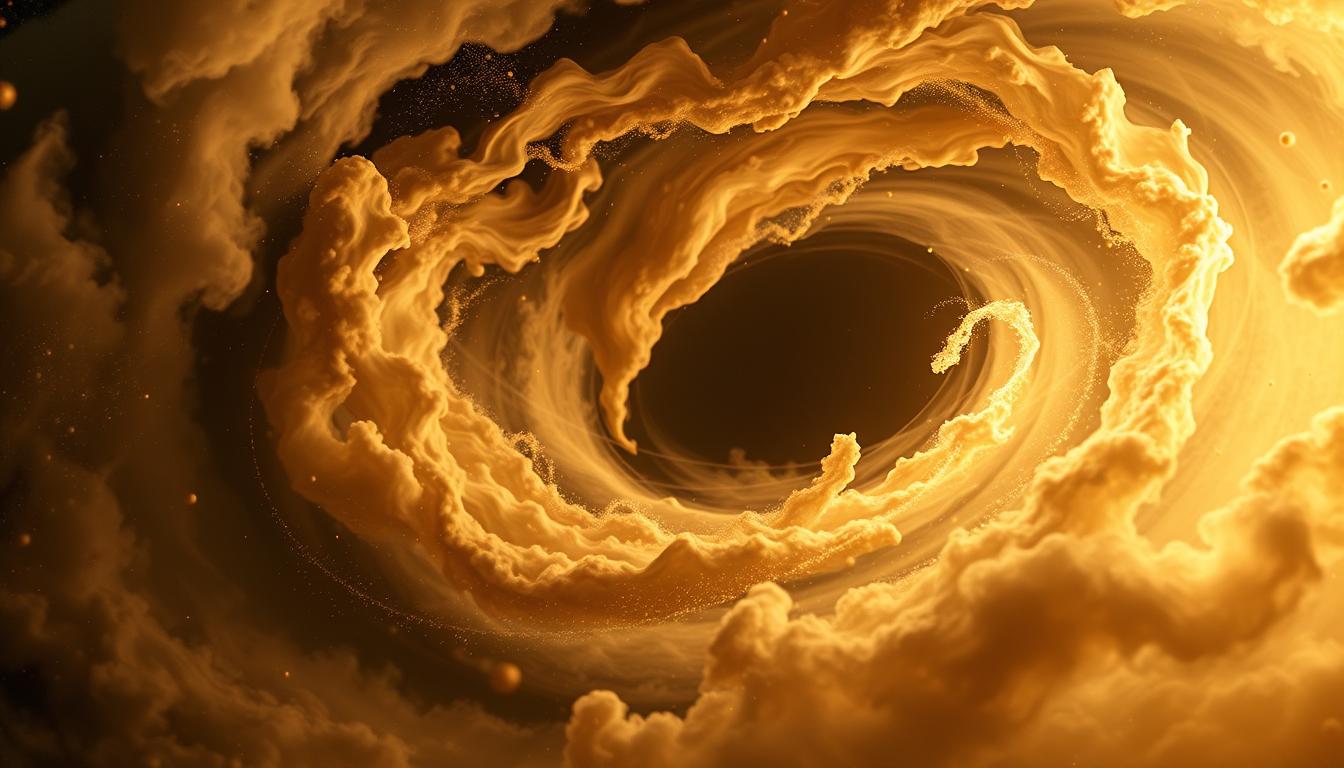
The Role of Gas, Dust, and Gravity in Planet Formation
When it comes to planet-making, gas, dust, and gravity are the dream team. Each one plays a big role in turning that spinning disk of stuff into actual planets. These elements work together to create diverse worlds, the exoplanets.
We covered the accretion phase that allows these grains of dust to gather together and form planetesimals. Later, as gravity gets stronger and they create a core, they turn into a proto-planet. As you can see, dust and gas are the keys to this process. Dust comes together, and clumps and gas help them to stick together with statistical force. Later, gravity comes in to get them bigger and stronger. The composition of these materials also plays a key role. Hydrogen and helium dominate the outer regions. This formed gas giants like Jupiter and Saturn. Closer to the center, heavier elements like metals and silicates created rocky planets like Earth.
This way of forming planets isn’t unique to our system. Similar processes likely occur in other star systems. Most likely, every planet started like this, going through a similar evolutionary process that living things have gone through on Earth.
Dissecting Protoplanetary Disks and Their Lifetimes
Another aspect of where gas and dust play a crucial role is in the formation of stars. Particularly the baby stars. A baby star is born and gets surrounded by swirling clouds of gas and dust. That’s a protoplanetary disk. It’s like the Sun’s messy nursery where all the planets, moons, and asteroids are about to be born.
These disks don’t last forever. Over time, they either form planets or get blown away by stellar winds and radiation. Scientists can see these disks around young stars in other galaxies. We can see baby pictures of stuff on our solar system. Cool, right?
Observational Evidence from Young Stars
One cool thing about the young stars and modern astronomy is that we can see it! Modern telescopes like ALMA have provided stunning images of protoplanetary disks. These observations also show us temperature gradients and the growth of dust grains over millions of years. Such data helps us understand how these disks evolve and eventually dissipate.
Studies show that most disks last only a few million years. During this time, planets begin to form, and the disk gradually loses its material. Rotation and turbulence affect this process. This process transports material across the disk.
Meteorites & Paleomagnetic Evidence: Decoding the Early Solar System
Okay, so we talk about ancient history billions of years ago out in space. We can do that by very different things. From our current telescopes looking into billions of years ago to studying the exoplanets we find. One way of doing this is by studying the meteorites and the paleomagnetic evidence on them. If you don’t know, meteorites are fragments of asteroids and other objects that were created in the early solar system. They tell us about the materials that were flying around back then, and sometimes even contain tiny grains that formed before the Sun did.
Paleomagnetism means studying magnetic patterns in ancient rocks. These patterns tell us how the solar nebula was spinning and cooling when everything was starting out. It’s like reading the fingerprints of the early universe. One key discovery comes from analyzing the magnetic fields in these ancient rocks. Paleomagnetic evidence clearly shows that the early solar system had a dynamic magnetic environment.
Also, we can age-date meteorites. Doing this gave us precise timelines for solar system events. These studies show that the belt formed rapidly within just a few million years. The composition of meteorites also links them to the asteroid belt. Many contain materials that match those found in asteroids.
Giant Planet Formation and Orbital Migration Insights
There is a type of planet that is sometimes called a giant planet and sometimes called a gas giant. The two good examples of this type of planet are Jupiter and Saturn. They didn’t just appear out of nowhere; they had a much more dramatic story of origin than their rocky cousins. These planetary titans formed farther out in the solar system, where there was a lot of ice and gas, and temperatures were cooler. Once they formed, they started to wander and found themselves in our solar system.
Understanding how they formed and how they might’ve changed lanes in the solar system is key to figuring out why things ended up where they are today. Their movements might have shaped the fates of smaller planets, stirred up asteroid belts, and even influenced Earth’s habitability. There are two main theories—core accretion and gravitational collapse—that try to explain how gas planets formed.
Core Accretion vs. Gravitational Collapse
The core accretion model suggests that giant planets start with a solid core of rock and ice. Over time (when their mass hits equal to about 10 Earth masses), this core attracts surrounding gas, leading to a rapid build-up of mass. This process is supported by evidence showing that gas giants formed within the first 4 million years of the solar system.
In contrast, the gravitational collapse theory proposes that giant planets form directly from collapsing gas clouds. This model explains the quick formation of massive bodies. It struggles to account for the rocky cores observed in planets like Jupiter.
Orbital Migration and Its Impact
Once formed, giant planets didn’t stay in one place. Orbital migration occurred as these planets interacted with their surrounding gas disks. For example, Jupiter is believed to have migrated inward to 1.5 AU (astronomical units) before moving outward to its current position at 5.2 AU.
This movement had massive effects on the solar system’s architecture. It influenced the distribution of smaller bodies and may have even cleared out early planetesimals. The timing of this migration, between 60 and 100 million years after the solar system’s formation, is supported by paleomagnetic evidence from meteorites.
Conclusion
Essentially, what is a solar nebula? A solar nebula is a dusty cloud from billions of years ago that started the formation of planets, stars, and everything in the cosmos. From floating gas to flaming stars, from pebbles to planets—our solar system came together piece by cosmic piece, thanks to the solar nebula.
Recent studies, like those analyzing paleomagnetic records in meteorites, provide a refined timeline for these events. They show that critical stages in the formation of our solar system occurred within the first few million years.
It’s wild to think that the Sun, Earth, and even your phone battery (thanks, lithium!) all trace back to the same leftover clouds from the Solar Nebula. Next time you look at the sky, just remember: you’re looking at the aftermath of one heck of a space party.
FAQ
How did the solar system begin its formation?
The process started with a massive cloud of interstellar dust and gas. Gravity caused this cloud to collapse, forming a rotating disk known as the solar nebula.
What role did gravity play in the early stages of star formation?
Gravity pulled material together, creating a dense core at the center. This core eventually became the Sun, while the surrounding disk formed planets and other bodies.
How do gas and dust contribute to planet formation?
Gas and dust provide the raw materials for building planets. Gravity pulls these elements together, forming cores that eventually attract more material.
What challenges remain in studying solar nebula models?
Scientists are still exploring how migration, material distribution, and disk lifetimes influenced the system’s evolution. Ongoing research aims to refine these theories.