Black holes are an essential thing in our universes, long-lasting bodies of dying stars, at least those which were capable in size of turning to a black hole. These holes are mostly black boxes to us, how they work, what’s inside of them, and what happens to the information that goes inside. These entities, have an immense gravitational pull that can bend light and distort space-time. It’s clear that they are extremely powerful in many senses but how many black holes are there in the vast universe? Do they pose a threat to us if there is like a bunch of them? And how do they come into existence? Also, what’s their lifespan?
Check this out: How Big are Black Holes?
I have to say, there is no definitive answer to determining how many black holes there are. There are a couple of reasons behind this. In this article, I will try to deep dive into this topic, give you an estimation of how many of them are there, and other related things.
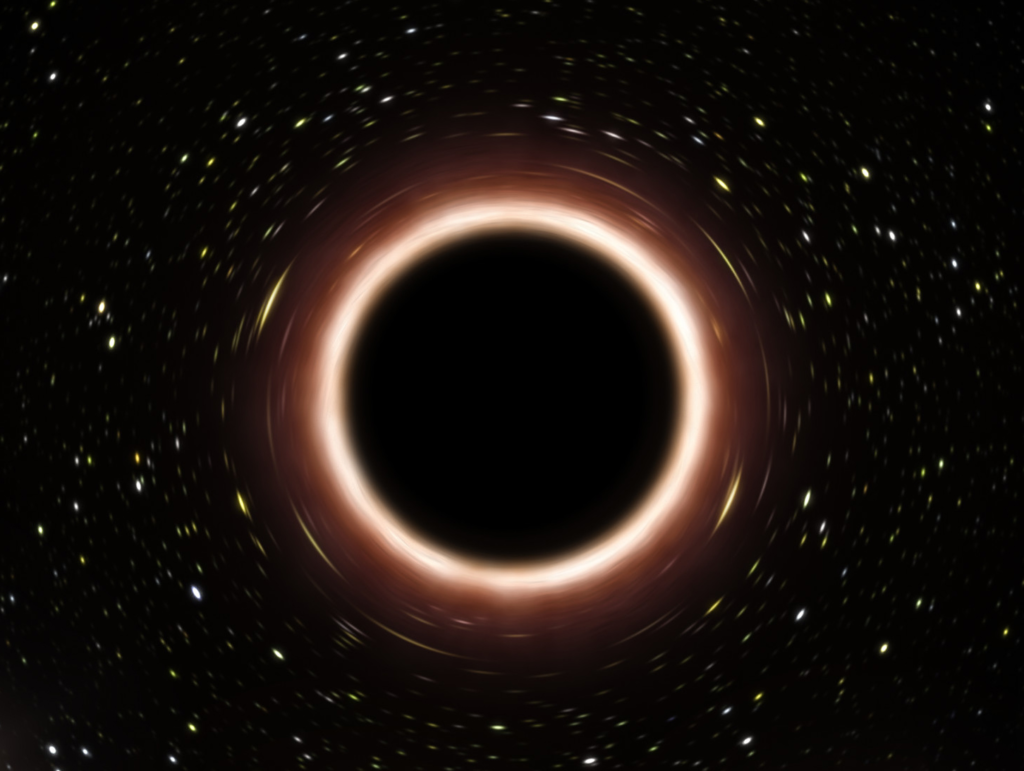
Understanding What a Black Hole Is
Black holes are extraordinary cosmic objects that possess an extreme gravitational force. Nothing, not even light, can escape their grasp. They are the aftermath of a collapse of a star in the universe. Not just a normal star, though. Not every star becomes a black hole, they have to be big enough to turn into one, so they are actually pretty rare compared to the number of starts that have lived and died out in the universe.
At its core, a black hole is a region in space where the gravitational pull is so intense that it creates a point of infinite density known as a singularity. This singularity has a surrounding line called an event horizon. The event horizon is basically the boundary of the black hole. Beyond this event horizon, nothing can escape the gravitational pull of the black hole.
The event horizon is often described as the “point of no return.” Once an object crosses this boundary, it is inevitably drawn into the black hole’s gravitational field. It disappears from our observable universe. The size of this event horizon boundary depends on the mass of the black hole. Larger black holes have larger event horizons.
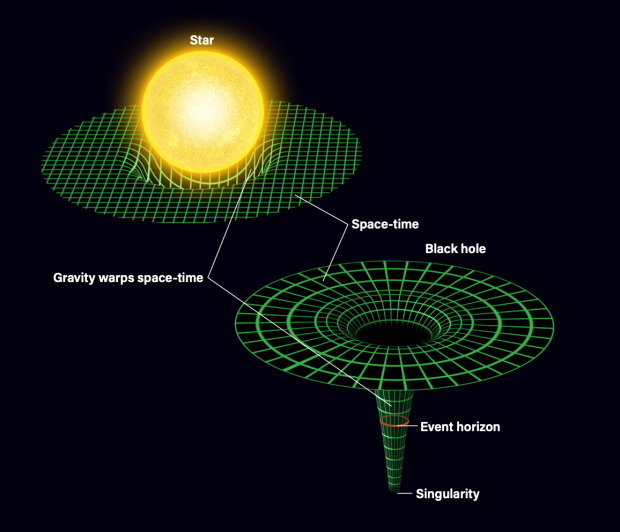
Conceptualizing Black Holes
One way to conceptualize a black hole is to imagine a massive star that has exhausted its nuclear fuel. Under the force of gravity, the star collapses inward, causing its core to become incredibly dense. If the mass of the core exceeds a certain threshold, the collapse continues until there is a singulary formation. This gives birth to the black hole as we know it. Also the certain threshold is known as the Tolman-Oppenheimer-Volkoff limit.
Another important characteristic of a black hole is its ability to warp space-time. According to Einstein’s theory of general relativity, mass, and energy curve the fabric of space-time. This creates what we perceive as gravitational forces. In the vicinity of a black hole, this curvature becomes extreme. That extreme curvature leads to the bending of light rays and the distortion of space itself.
How Are Black Holes Formed?
Black hole formation happens through various processes. It depends on the mass of the object and the conditions under which it undergoes gravitational collapse. Depends on these conditions, a black hole might form or might not. When it forms, its size also depends on these conditions.
Stellar Evolution and Black Hole Formation
One common pathway to black hole formation is through the evolution of massive stars. Massive stars, which have a mass several times greater than that of our Sun, go through a series of stages in their lifecycle.
Initially, massive stars burn hydrogen in their cores through nuclear fusion. Through this burning, they generate immense amounts of energy that counteract the inward force of gravity. However, as they exhaust their hydrogen fuel, these stars begin to fuse heavier elements, such as helium, carbon, and oxygen.
Eventually, a massive star reaches a point where it can no longer sustain nuclear fusion. At this stage, gravity takes over, causing the star to collapse under its own weight. The core of the specific star becomes incredibly dense which leads to the formation of a neutron star. If the mass is high enough, it could also form a black hole.
When the collapsing core of a massive star exceeds the Tolman-Oppenheimer-Volkoff limit (approximately 3 times the mass of our Sun), the gravitational collapse continues until a singularity is formed, resulting in the birth of a stellar black hole. These black holes are typically a few times to several tens of times more massive than our Sun.
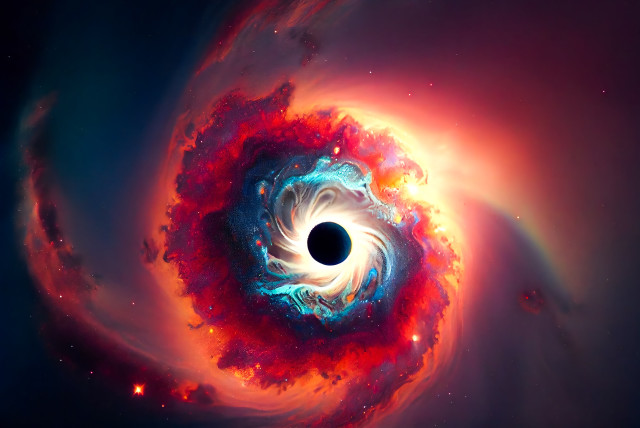
Supermassive Black Holes and their Origins
In addition to stellar black holes, there are also supermassive black holes. These ones are millions or even billions of times more massive than our Sun. We are still researching the formation mechanisms of a supermassive black hole, but there are several proposed theories.
One theory suggests that a supermassive black hole form through the gradual accretion of matter onto a smaller, seed black hole. This accretion process occurs as nearby gas and dust are drawn into the gravitational well of the black hole, gradually increasing its mass over time.
Another theory proposes that they form through the direct collapse of massive gas clouds in the early universe. These gas clouds, known as primordial black hole seeds, would have existed in the early stages of the universe. They could have rapidly grown into a supermassive black hole through subsequent accretion.
As I said, we still don’t know for sure how this type of black hole comes into existence. Scientists are still searching but I don’t think there will be an answer soon. Mostly because these are so big and hard to come by in our present universe, we can’t just see how they form in the near past. They happened billions of years ago in the cases we know.
Direct Collapse Black Holes
While stellar evolution and accretion processes explain the formation of many black holes, there is also a possibility of direct collapse of black holes. Direct collapse of a black hole is theorized to form from the collapse of massive gas clouds without the intermediate stage of a star.
These gas clouds, which have high density and low angular momentum, can potentially bypass the formation of a protostar and directly collapse into a black hole. We believe that there are various factors that trigger this process. The presence of a nearby massive object or the interaction of multiple gas clouds could be some examples.
Direct collapse black holes are thought to be the progenitors of the earliest supermassive black holes observed in the universe. Studying them can provide insights into the early stages of galaxy formation and the evolution of the cosmos.
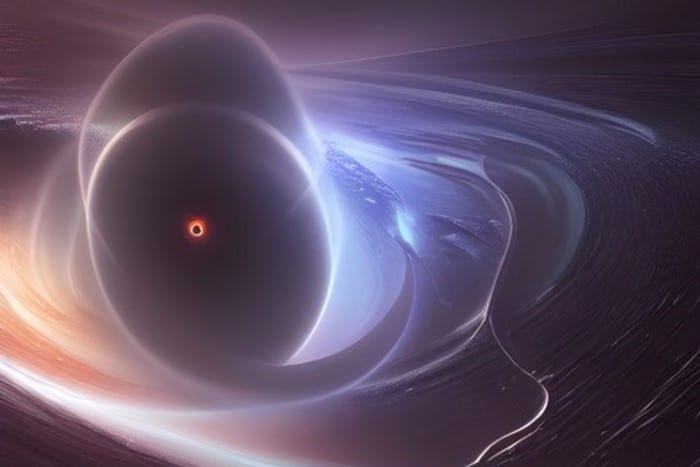
Methods of Detecting a Black Hole
Detecting a black hole is a complex task due to their nature and that they don’t emit visible light. However, scientists have developed several ingenious methods to indirectly observe and study these enigmatic objects.
Gravitational Waves
One groundbreaking method of detecting black holes is through the observation of gravitational waves. Gravitational waves are essentially ripples in the fabric of space-time. The cause of gravitational waves is the acceleration of massive objects. When two black holes merge, they create intense gravitational waves that propagate through the universe.
In recent years, the advancement of gravitational wave detectors allows scientists to directly observe these gravitational waves. Some gravitational wave detectors are the Laser Interferometer Gravitational-Wave Observatory (LIGO) and the Virgo detector. By analyzing the patterns in the detected waves, scientists can infer the presence and characteristics of the merging black holes.
Gravitational wave detection not only provides evidence for the existence of black holes but also allows scientists to determine their masses, spins, and even their distances from Earth. This method has opened up a new era in black hole astrophysics and has led to numerous groundbreaking discoveries.
X-Ray Emissions
Black holes, particularly those that are actively accreting matter, can emit X-ray radiation. As matter falls into a black hole’s gravitational well, it forms an accretion disk around the black hole. The intense gravitational forces and the friction within this disk generate tremendous heat, causing the material to emit X-rays.
Satellites equipped with X-ray detectors, such as NASA’s Chandra X-ray Observatory, are crucial in observing and studying these X-ray emissions. By analyzing the X-ray spectra and light curves, scientists can have an understanding of the properties and behavior of a black hole. They can understand their mass, accretion rate, and the dynamics of the surrounding matter.
X-ray observations have been instrumental in identifying and characterizing black hole candidates in binary systems, where a black hole is in a close orbit with a companion star. These observations provide valuable data for understanding the accretion processes and the interactions between black holes and their stellar companions.
Observing Effects on Nearby Objects
Another indirect method of detecting a black hole is by observing their effects on nearby objects. Due to the extremely dense gravitational force a black hole has, it can significantly influence the motion and behavior of nearby stars and gas clouds.
By carefully monitoring the orbits of stars in binary systems or globular clusters, scientists can detect the presence of an unseen object with a high mass. This indicates the presence of a black hole. These observations rely on precise measurements of the Doppler shifts or positional changes of these objects over time.
For example, if a star or a planet is orbiting around a black area in the universe without anything around it, that is a good sign that there might be a black hole in the middle of it. The ravitational pull of a black hole can cause the distortion and stretching of light from background stars. This leads to a phenomenon known as gravitational lensing. This lensing effect can be used to indirectly infer the presence and mass of a black hole.
By combining various observational techniques, such as optical telescopes, radio telescopes, and high-resolution imaging, scientists can gather valuable data on the effects of black holes on their surroundings, helping to confirm their existence and study their properties.
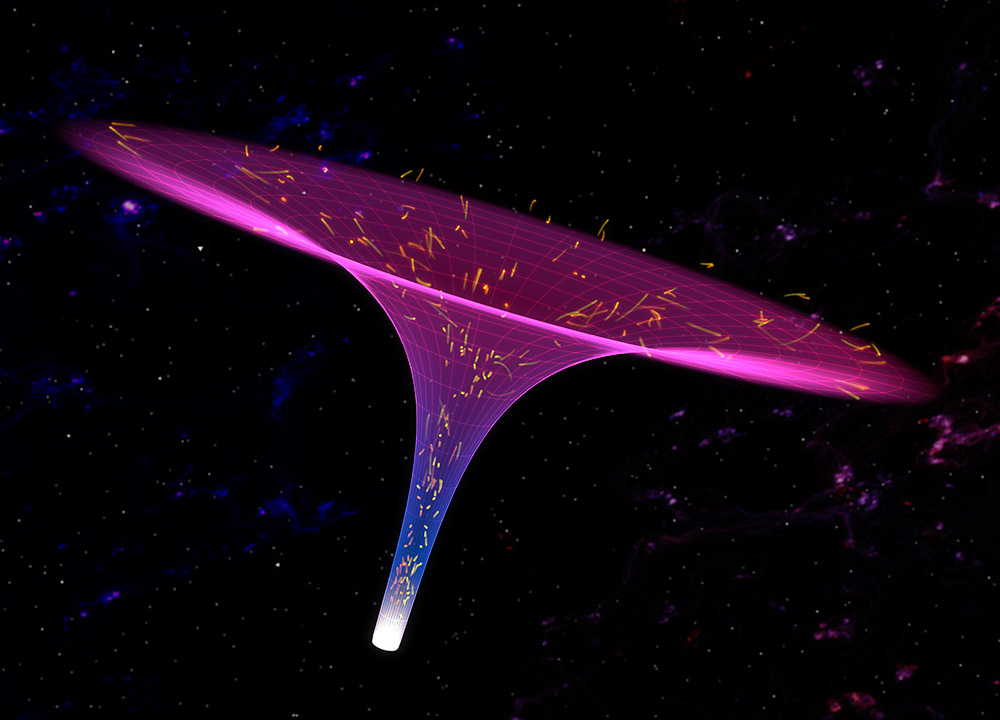
Estimating the Number of Black Holes
As I mentioned in the beginning of the article, estimating how many black holes there are is essentially impossible. We can’t just come up with a number that will be correct, that will be ascientific. I mean, this is at least with our current technology. Maybe in millions of years and humans aren’t exctint, we may come up with an exact number but that’s still quite hard, if not impossible.
However, we can make assumptions, right? By using some data and other stuff we already know, we can have an estimation within a range.
Number of Stellar Black Holes
Stellar black holes are formed from the remnants of massive stars that have had gravitational collapse. These ones’ mass ranges from a few times to several tens of times that of our Sun. Estimating the number of stellar black holes in the universe involves understanding the population of massive stars and their subsequent evolution.
Scientists study the demographics of massive stars, their birth rates, and their lifetimes to estimate the number of stellar black holes that can form. By observing the distributions of stars in different regions of our galaxy and other galaxies, astronomers can infer the number of massive stars that eventually evolve into a stellar black hole.
Additionally, studying binary star systems, where a black hole is in a close orbit with a companion star, provides valuable insights into the population of stellar black holes. By observing the gravitational interactions and mass transfer between the black hole and its companion, scientists can estimate the frequency of such systems. This can also help to estimate to see how many stellar black hole there are.
Number of Supermassive Black Holes
Supermassive black holes sit at the very centers of galaxies. They can have masses ranging from millions to billions of times that of our Sun. Estimating the number of supermassive black holes requires a combination of observations of galaxies, theoretical models, and statistical analyses.
Observations of galaxies across the universe provide insights into the presence and characteristics of supermassive black holes. Studying the motion of stars and gas in the galactic centers, and the emission signatures from accretion disks, we can sense the presence and mass of supermassive black holes.
Theoretical models of galaxy formation and evolution also play a crucial role in estimating the number of supermassive black holes. These models consider the growth of black holes through accretion processes, mergers of galaxies, and the interplay between black holes and their host galaxies.
By combining observational data with theoretical models, scientists can derive statistical estimates of the population of supermassive black holes in the universe. These estimates provide valuable insights into the prevalence and distribution of these cosmic giants, contributing to our understanding of galaxy evolution.
Total Estimated Black Holes in the Universe
Estimating the exact number of black holes in the universe is extremely challenging and basically impossible. However, by combining the estimated populations of stellar black holes and supermassive black holes, we can have a rough estimate of the total number.
Based on current knowledge and observations, scientists estimate that there could be millions to billions of stellar black holes in our galaxy alone. Extrapolating this to the billions of galaxies in the observable universe suggests that the total number of stellar black holes could be staggering.
Similarly, considering the prevalence of supermassive black holes in galactic centers, it is believed that there could be millions to billions of these massive black holes in the universe.
It’s important to note that these estimates are subject to ongoing research, refinements in observational techniques, and advancements in theoretical models. As our understanding of black holes and the universe continues to deepen, our estimates of their quantity will become more precise.
Ongoing Research and Discoveries
Ongoing research and discoveries in the field of black hole astrophysics are continuously expanding our knowledge and deepening our understanding of these enigmatic cosmic objects.
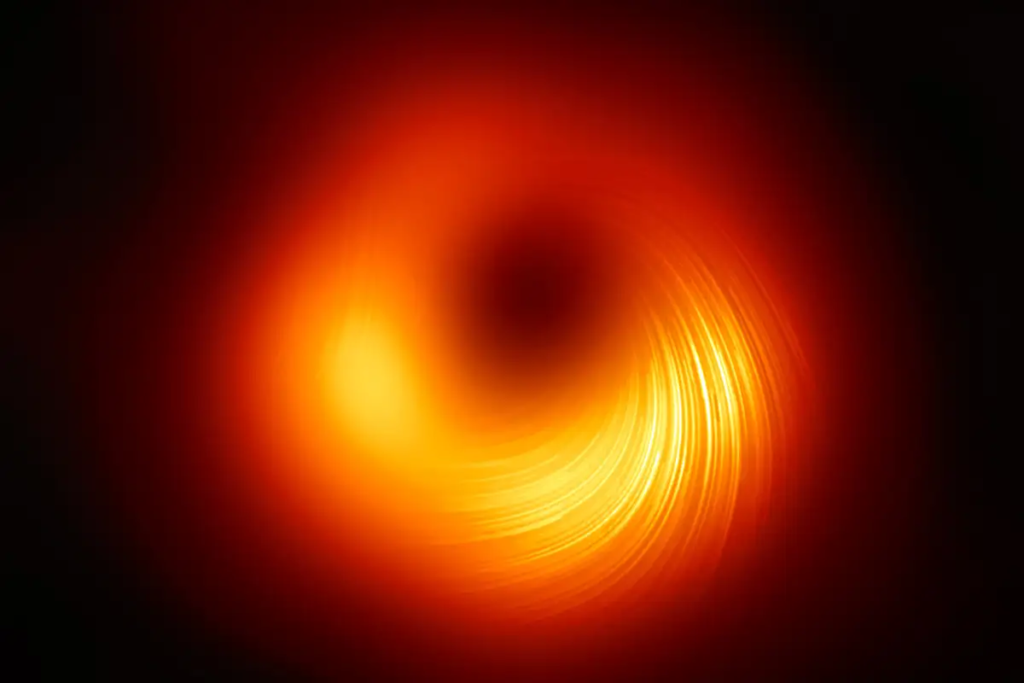
Advanced LIGO and Virgo Collaborations
The Advanced Laser Interferometer Gravitational-Wave Observatory (LIGO) and the Virgo detector have revolutionized the field of astrophysics by directly detecting gravitational waves. These observatories have already made groundbreaking discoveries, including the first direct detection of gravitational waves from the merger of two black holes.
The ongoing research and continuous improvements in the sensitivity of these detectors are expected to unveil more black hole mergers and provide valuable data for studying the properties and populations of black holes.
Event Horizon Telescope and the First Black Hole Image
In April 2019, the Event Horizon Telescope (EHT) collaboration made history by capturing the first-ever image of a black hole. By combining data from a global network of radio telescopes, the EHT team produced an image of the supermassive black hole at the center of the galaxy M87.
This achievement not only confirmed the existence of black holes but also provided visual evidence of the event horizon, offering insights into the structure and properties of these cosmic entities. Ongoing observations and analysis by the EHT collaboration continue to deepen our understanding of black holes and their immediate surroundings.
Future Space Missions
Several upcoming space missions are dedicated to studying black holes and unraveling the mysteries of the universe. The European Space Agency’s Athena mission, set to launch in the 2030s, aims to study the hot gas and X-ray emissions from black holes and other cosmic objects. It aims to provide valuable insights into their formation and evolution.
NASA’s James Webb Space Telescope will also greatly contribute to black hole research by observing the early universe and studying the formation and growth of galaxies. This includes the role of supermassive black holes in their evolution.
These future missions hold the promise of expanding our knowledge and shedding light on the quantity, nature, and behavior of black holes. It wil contribute to our understanding of the universe on a grand scale.
In conclusion, ongoing research and discoveries in the field of black hole astrophysics are continuously pushing the boundaries of our understanding. From the detection of gravitational waves to the imaging of black holes, these advancements are allowing us to explore the quantity and nature of black holes in unprecedented ways.
As technology and observational techniques continue to improve, we can expect even more exciting discoveries and a deeper understanding of these cosmic giants.