Dark matter has been a mysterious object for decades for us. We’ve been trying many different things to detect dark matter with different dark matter experiments, whether directly or indirectly, but there hasn’t been a lot of success. Some of the dark matter experiments have brought us closer, though. After all, 85% of all matter in the universe is dark matter. We should be able to see or detect it one way or another.
Check this article out, too: Dark Mattter vs Dark Energy: What’s The Difference?
Most of these dark matter experiments use particle physics experiments. Fermilab hosts some of the famous dark matter experiments, for example. Another one is the SuperCDMS experiment, located in a Canadian mine. The lab above the mine is a clean, state-of-the-art space, unlike the mine below. They remove earthly particles to detect dark matter’s presence. Despite advanced technology, they often find no signal.
From the LHC to sophisticated models, scientists are narrowing down where dark matter might be. This is a global effort, with many scientists and labs working together. The DOE Office of Science and the NSF’s Particle Astrophysics and Nuclear Physics Programs are key players in dark matter experiments. Let’s look at the history of dark matter detection with dark matter experiments. See what we have found so far and what the future holds.
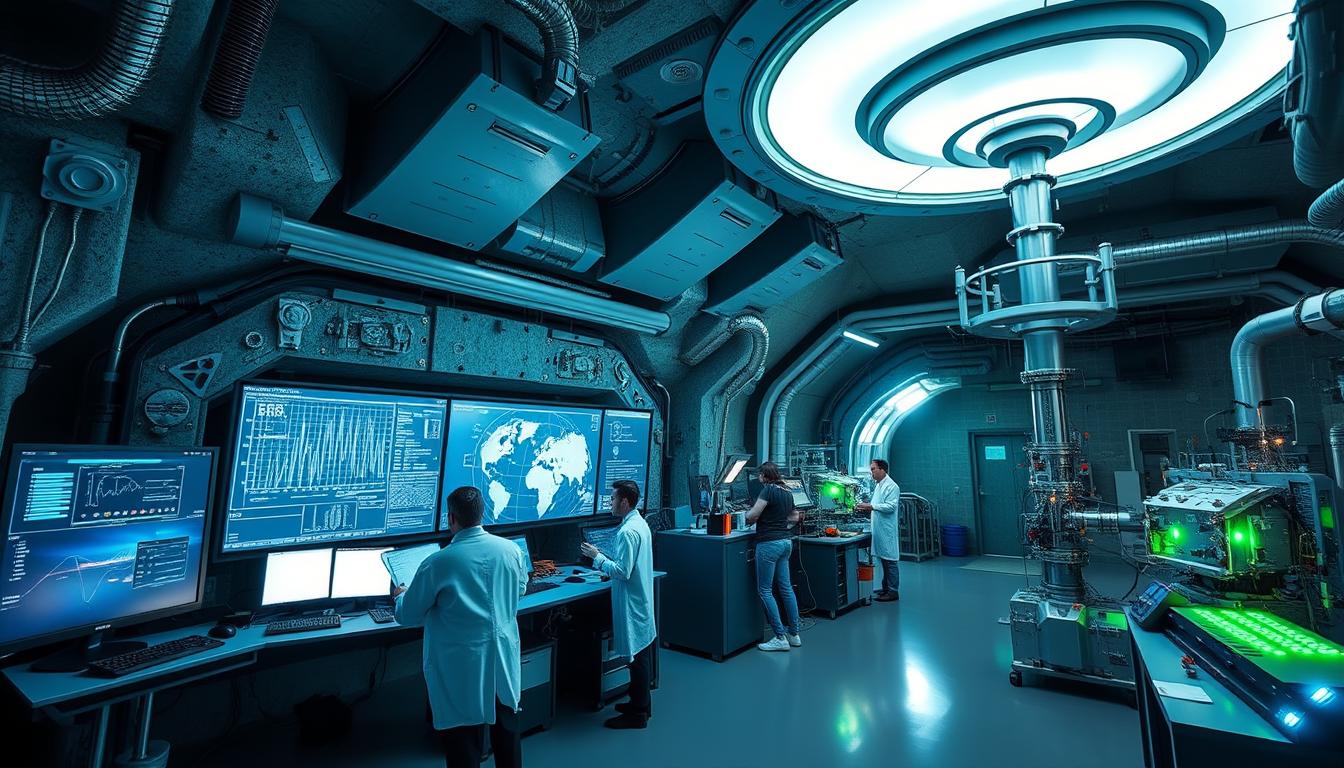
The Discovery and Hypotheses of Dark Matter
Dark matter’s discovery started with a mystery: the mass of big objects didn’t match what we could see. Scientists found that something unseen was pulling on them, leading to the idea of dark matter—about 85% of the universe’s mass! Dark matter doesn’t reflect light, but it affects the universe’s shape and growth. The Bullet Cluster and galaxy rotation are two of the most prominent studies that show its existence. Even Type Ia supernovas help us understand how dark matter affects the universe’s expansion.
Over the years, as we know more and more about dark matter, the research has intensified, and there is a lot more stuff happening than it did two decades ago. How has dark matter research advanced over the years? Teams from places like the University of Chicago and Fermilab have made big changes in dark matter research. They created a system that resembles cosmic detectives to scan for dark matter signals. Cryogenic Dark Matter Search (SuperCDMS I mentioned above), part of Fermilab, is one of those searches that’s quite important and is quite close to understanding dark matter. Their goal is to find even the smallest signs of dark matter using sophisticated and sensitive technology.
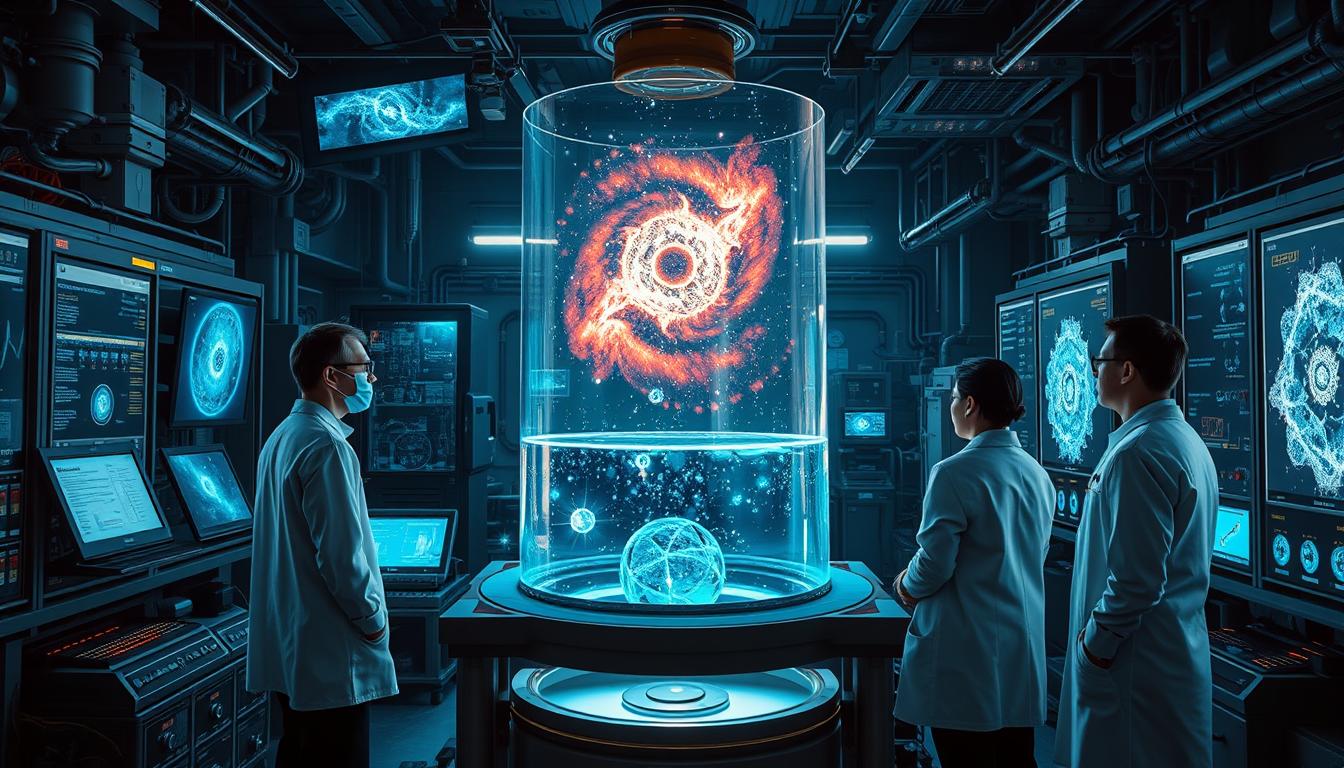
Fermilab’s Efforts to Detect Dark Matter
When talking about dark matter research, I have to open a separate heading for Fermilab. Fermilab is a top name in high-energy physics, leading in dark matter experiments. They have many exciting projects to try and detect dark matter. The Cryogenic Dark Matter Search is one of those key projects at Fermilab. It’s set up deep in mines for the best WIMP detection experiments. The Darkside experiment also uses different materials to find WIMPs, which are thought to be a big part of dark matter.
Fermilab, as you can understand from the name, is a laboratory. So their only goal isn’t to try and find dark matter. It does a lot of stuff. As part of their dark matter research, they also work with schools in the Chicagoland area. This helps create a lively research scene and bring fresh minds to the table. It’s not just about growing science; it’s also about training the next scientists in dark matter experiments. Fermilab keeps bringing in new ideas and young scientists to the field.
One of the things that Fermilab does is use advanced tools to try to reach the neutrino floor. This is a point where neutrinos might hide dark matter signals. Getting past this point is key to finding out more about our universe. They also do WIMP detection experiments as part of their journey. They try to hit it from every angle possible. I will explain why they are doing WIMP experiments below. Many believe that WIMPs may be dark matter.
The Principles and Challenges of Direct Dark Matter Detection
There are two main dark matter detection methods, direct and indirect. Direct is the best detection method to prove dark matter with tangible results. However, since dark matter doesn’t reflect or emit light, directly detecting it is extremely hard. When we are trying to detect dark matter directly, we assume Earth moves through a dark matter halo. This idea uses special equipment to detect dark matter. High-energy physics experiments aim to understand this with different types of dark matter detection experiments, which are different from some others we do.
Direct dark matter research focuses on how dark matter interacts with regular matter. Theoretical predictions suggest dark matter particles could be very light or very heavy. This wide range makes it hard for one detector to find all of them. Experiments like Xenon1T have set limits on dark matter properties. They use noble gases to detect rare interactions between dark matter and regular matter.
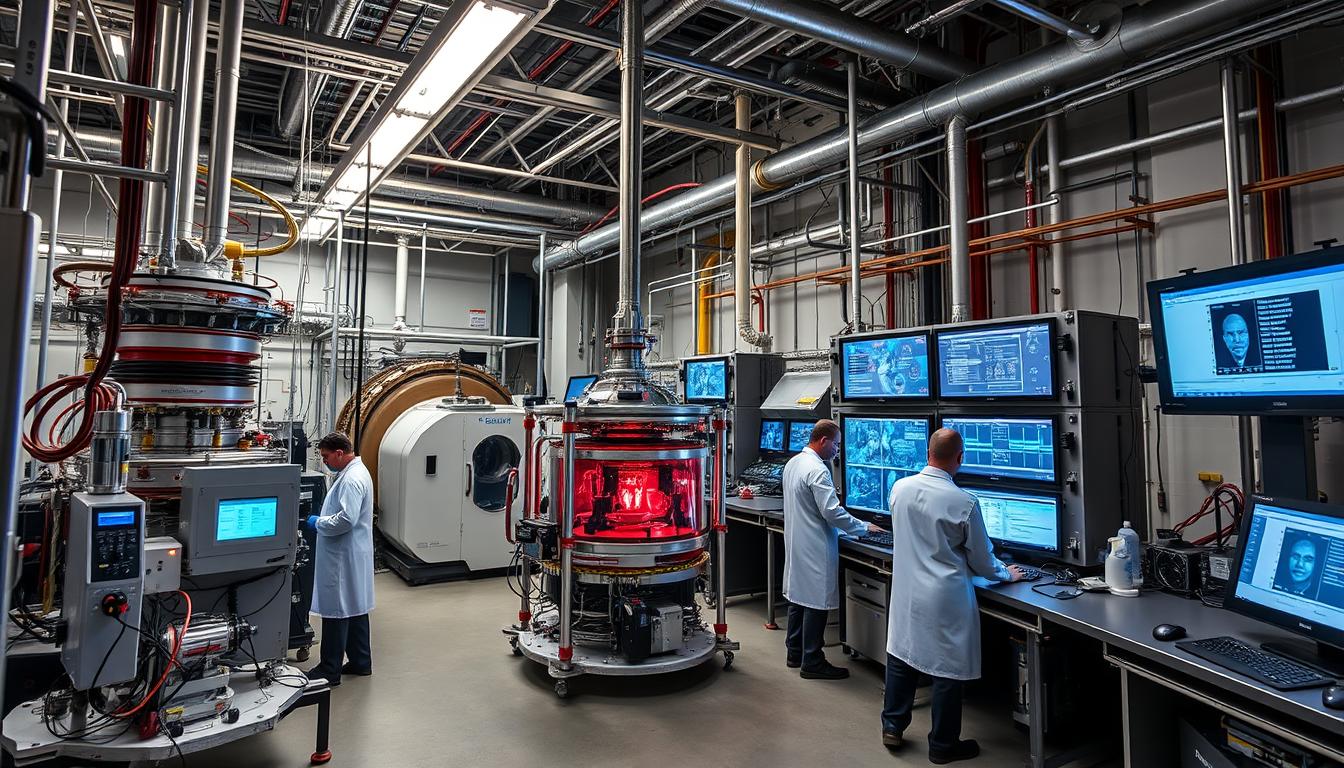
What Could Dark Matter Be?
Alright, we try to detect and understand dark matter – but what is dark matter, or what could it be? We have some hypotheses, but none of them could be true, as well; they are only theories. Each has different ways to detect it and different characteristics.
Axions
Axions are hypothetical, very light particles like tiny, shy ghosts of the particle world. They are super lightweight, almost weightless, and don’t interact much with anything else—no charge, push or pull. The idea comes from a puzzle in physics about why the universe doesn’t wobble like a spinning top. Axions, if they exist, could fix this problem and at the same time fill up the universe as invisible, floating particles.
Scientists hope to find them by watching how they turn into photons in magnetic fields. The Axion Dark Matter Experiment (ADMX) is one of the tools used for this. They use powerful magnets to create resonances, hoping to “hear” the axions convert into faint radio waves. It’s like tuning a radio to pick up the weakest, most elusive signal ever.
Weakly Interacting Particles (WIMPs)
WIMPs (Weakly Interacting Massive Particles) are like the heavyweight champions of the dark matter candidates. These particles are much heavier than axions and interact weakly with other matter, which makes them tricky to catch. Scientists like WIMPs because they naturally fit into many ideas about the universe’s birth during the Big Bang. WIMP detection experiments look for their rare interactions with regular matter.
Scientists go deep underground into mines or mountaintops to catch a WIMP, far away from noisy cosmic rays. Experiments like LUX-ZEPLIN use giant tanks filled with liquid xenon. If a WIMP bumps into a xenon atom, it might cause a tiny flash of light. It’s like watching a calm pond and hoping to see a ripple from a stone you didn’t throw. Fermilab is leading the way in this research. Their work is at the forefront of dark matter research.
Sterile neutrinos
Neutrinos are already strange—tiny, neutral particles that pass through you by the trillions every second. Sterile neutrinos are their even quieter cousins. These particles don’t interact with anything except gravity. They’re like shadows of the already ghostly neutrinos. Sterile neutrinos could explain some mysteries, like the strange way galaxies clump together. To look for them, scientists study the behavior of regular neutrinos in experiments like MiniBooNE and IceCube. They hope to catch a sterile neutrino, leaving a faint, indirect trace, like a whisper in a loud room.
Primordial Black Holes: Composite Dark Matter
Not all dark matter has to be tiny particles. Some scientists think dark matter could be primordial black holes, born moments after the Big Bang. These black holes are different from the ones formed by dying stars. They’re small—some as tiny as a city—and they could act as gravitational anchors for galaxies.
Astronomers use telescopes like Hubble and experiments like LIGO to hunt for these ancient monsters, which detect ripples in space-time. If a primordial black hole passes in front of a distant star, it might bend the star’s light—a trick called gravitational lensing. It’s like spotting a magnifying glass moving through a field of fireflies.
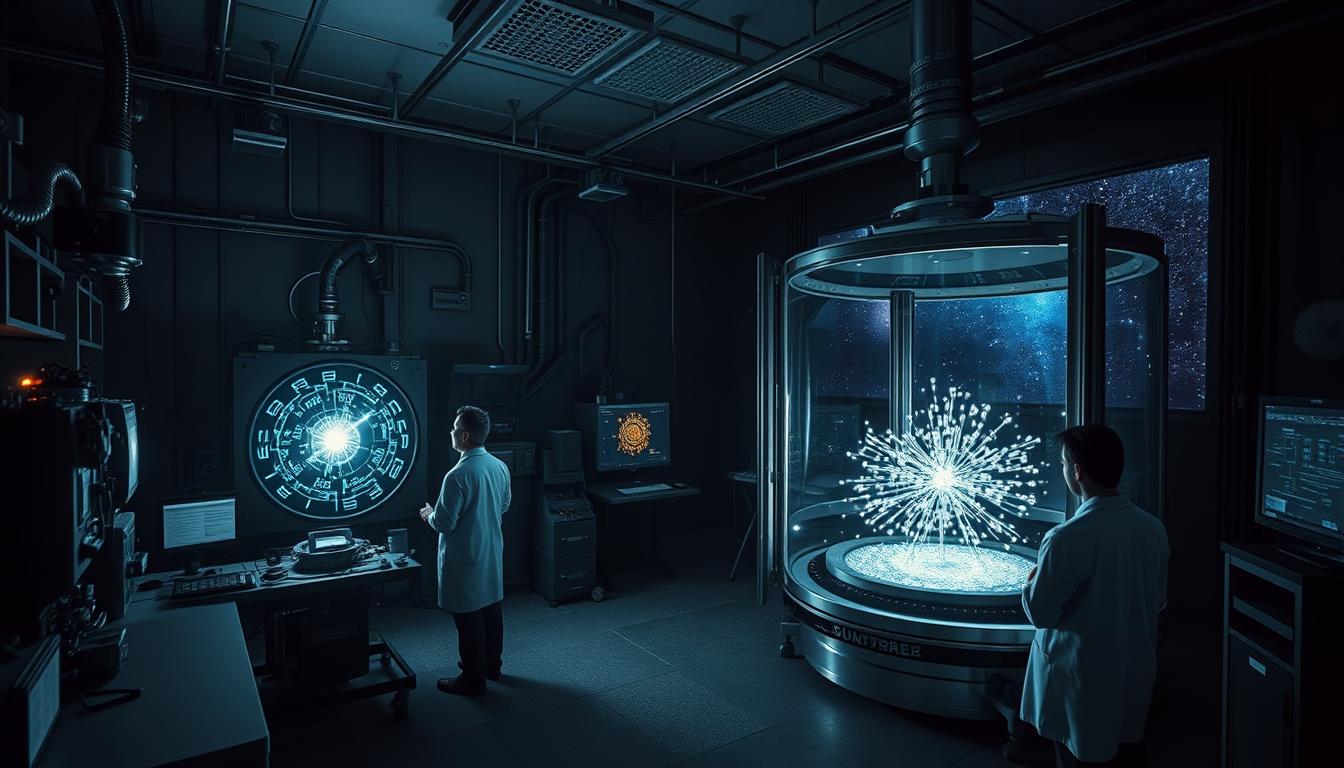
Dark Matter Experiments
Searching for dark matter is like searching for a needle in a cosmic haystack. I am sure you got that by now. That’s why if you want to find and detect dark matter, you need to be innovative and create different and unusual methods. There are some of these methods that we use quite frequently; each is like a different kind of trap. Let’s explore four of them: Cryogenic Crystal Detectors, Noble Gas Scintillators, Crystal Scintillators, and Bubble Chambers.
Cryogenic Crystal Detectors
Imagine holding a crystal so cold it’s just a whisper away from absolute zero. If a dark matter particle bumps into the crystal, it will cause the tiniest vibration—like a snowflake landing on a frozen pond. These detectors are designed to catch those subtle shivers. The crystals, often made of materials like germanium or silicon, are chilled to temperatures near -273°C. At this temperature, even the smallest energy change stands out. Experiments like CDMS (Cryogenic Dark Matter Search) use these detectors in deep underground labs to block out noisy cosmic rays. It’s like listening for a pin drop in a completely silent room.
Noble Gas Scintillators
Noble gases like xenon and argon have a special talent: they glow when struck by particles. Large tanks of liquid noble gas are placed deep underground in these detectors. If a dark matter particle collides with a gas atom, it creates a tiny flash of light and a trail of electrons. Experiments like XENONnT and LUX-ZEPLIN use sensitive cameras to catch these faint signals. The glow is incredibly weak, so the setup must be shielded from any other sources of light or radiation. It’s like waiting for a firefly to blink in the darkest night.
Crystal Scintillators
Crystal scintillators work a lot like noble gas detectors, but they use solid crystals instead of liquid. When a particle hits the crystal, it creates a spark of light. These detectors are excellent at distinguishing between different kinds of particles based on how they interact with the crystal. One famous experiment using crystal scintillators is DAMA/LIBRA. It looks for seasonal changes in dark matter signals, which might happen as Earth moves through the galaxy.
Bubble Chambers
Bubble chambers sound like something from a science fiction novel, but they’re real. These detectors use a tank filled with superheated liquid, kept just below its boiling point. When a particle, possibly dark matter, passes through, it leaves a tiny trail of bubbles. The beauty of bubble chambers, like the ones used in the PICO experiment, is their ability to ignore false alarms. They’re sensitive only to certain types of collisions. Scientists watch the bubbles grow and study them, like detectives examining clues at a crime scene.
Conclusion
We’ve been searching for dark matter with a variety of dark matter experiments for decades now. So far, we’ve made good progress, but not enough to have the first evidence of dark matter. I think it’s going to take another couple of decades until we have the first direct detection of dark matter. Mainly because our technology isn’t developed enough to do that.
Some dark matter experiments like DAMA/LIBRA reached a high detection level, but others like ANAIS have questioned these findings. So, there is always a counterargument to a positive detection one way or another.
Dark matter makes up 85% of the universe’s matter. It greatly affects how galaxies form and the universe’s structure. That’s why it’s important to understand dark matter and focus on what we can do with it once we find the first direct evidence. Our current thoughts that axions, WIMPs, or something else may be dark matter are pure theories. Some of the dark matter techniques, like bubble chambers, maybe the answer to turn that into a reality.
FAQ
What exactly is dark matter?
Dark matter is invisible because it doesn’t reflect or absorb light. We know it exists because it affects how galaxies move and bend light. This is seen in how galaxies spin and through gravitational lensing.
How do we know dark matter exists?
We can’t see dark matter, but we know it’s there. This is because galaxies move faster than they should, and the universe’s background radiation shows extra mass. These signs point to dark matter’s presence.
How does Fermilab contribute to the search for dark matter?
Fermilab is a leader in finding dark matter. They run experiments to detect dark matter particles. They use special technologies and methods to search for these particles.
Can you explain what axions are and how we search for them?
Axions are tiny particles that might make up dark matter. Scientists use strong magnetic fields and sensitive detectors to find them. This is done in experiments like the Axion Dark Matter Experiment (ADMX).
What role do experiments like the Large Hadron Collider (LHC) play in dark matter research?
The LHC at CERN collides with particles at high energies. This might create dark matter particles if they exist within the accessible energy range. Finding these signals could give us important information about dark matter.
What are the main challenges in detecting dark matter?
Detecting dark matter is hard because it interacts very weakly with normal matter. We need very sensitive detectors to be able to tell dark matter signals from background noise. Dark matter could have many different masses and interactions, making it even harder to find.